Research Activities
Liebig's law of the minimum provides a fundamental basis for understanding biological regulation: how the biological regulations of some core life processes (e.g., metabolism, assimilation) at the levels of cells, molecules and ecosystems are influenced by the availability of "limiting resources", and conversely what are the biological determinants for the bioavailability of essential mineral elements that are often limiting in most ecosystems.
Liebig's law is originally developed in agricultural
sciences, stating that growth is regulated not by total amount of resources,
but by the scarcest resource i.e., limiting factor. This concept is eminently
applied in agricultural practices and fertilizer industries to get eloquent
control on plant or crop growth. However, a general principle can be formed
based on this idea which underlies the process of regulation from molecules to
ecosystems, allowing us to conceive existence of a limiting factor critical to biological regulation. Probing how this
general principle works under different conditions and at different scales is
fundamental to regulatory mechanisms. We have observed that a suite of
regulatory mechanisms can emerge under the constraints of limiting
factors.
Working research problems
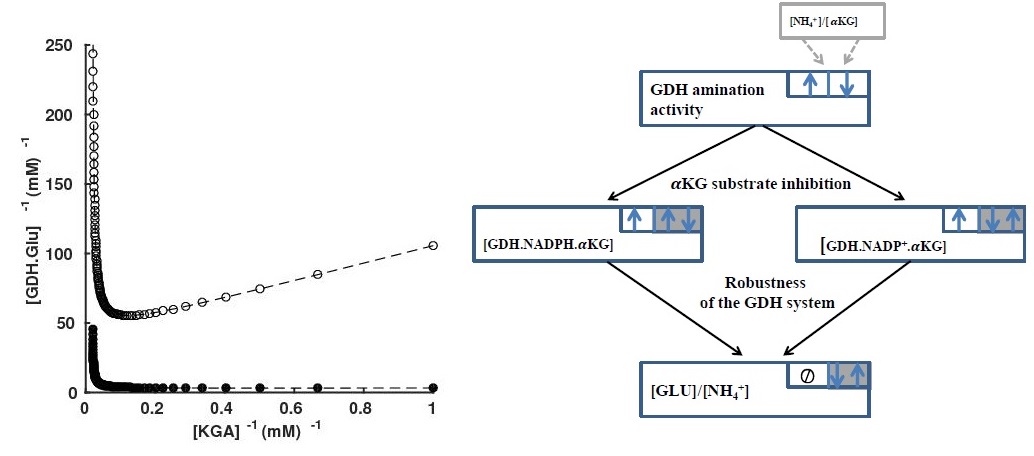
A kinetic mechanism underlying the robustness of the GDH ammonium assimilation system
- Nitrogen assimilation and
detoxification
Nitrogen is an essential
mineral element for the biosynthesis of all amino acids and nucleotides and
acts as limiting factor in most
biosystems. However, nitrogen can also have toxic effects with its ammonium
form generated as a by-product of amino acid metabolism. Hepatic cells play
critical role for detoxifying ammonium and for maintaining ammonium homeostasis
in systemic circulation (i.e.,
normal range 10-40 micro-mole per litre). But this same ammonium can also be an essential
input in the synthesis of glutamate and glutamine-primary donors of amine and
amide groups to most macromolecules. How the systemic ammonium homeostasis is
maintained by balancing competing processes of detoxification and amino acids
biosynthesis in hepatic cells remains elusive. I am targeting to unfold this
mystery, examining how the N-assimilation maintains robust input-output ratios
in response to large-extent variation of ammonium and alpha-keto glutarate
substrate which are essentially adjusted according to the N-demand. I also
address particularly glutamate dehydrogenase (GDH) enzyme dynamics. Over
activity of GDH causes hyperinsulinism/hyperammonemia
syndrome (HI/HA), resulting in recurrent hypoglycaemia in early infancy. This problem is tackled with
systems biology and biophysical approaches combined with 'molecular dynamic
simulation' tools and the available protein
data bank (PDB) information.
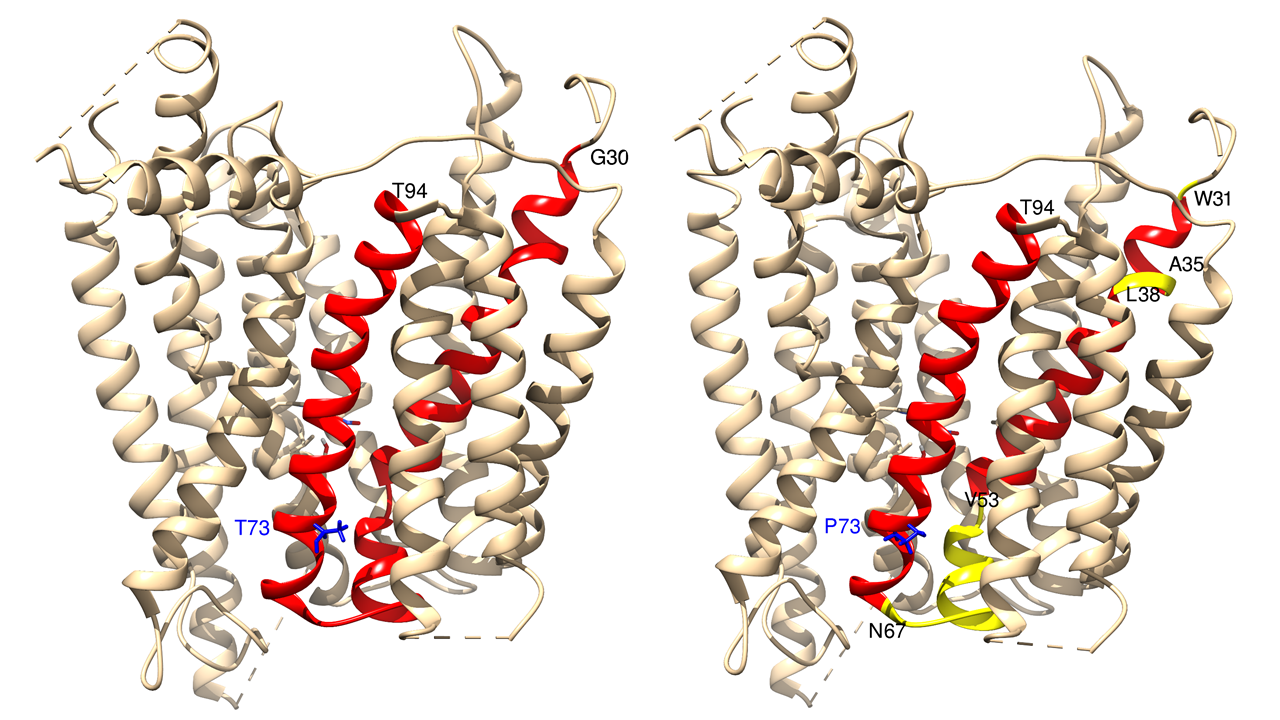
Nitrate transporter NRT1.1 rigidity analysis for identifying allosteric communication pathways
- Nitrate signaling and uptake
mechanisms in plants
Nitrate is most common bioavailable form of
nitrogen taken up by the plants and microbes. To be assimilated, it has
converted into ammonium by nitrate and nitrite reductase and then into amino
acids by enzymes such as glutamate synthase. In addition to being an essential
nutrient, nitrate also serves as signaling molecule. Out of several identified
nitrate transporter proteins (i.e., NRT1 and NRT2 families), NRT1.1 has
recently been shown to play the role of transceptor.
This interesting molecule can sense nitrate availability and then adjust the
primary nitrate response (PNR) by changing nitrate binding affinities. It has
been demonstrated recently that NRT1.1 has biphasic
toggle-switch type response to nitrate availabilities; at low nitrate, high
affinity mode of transport is ON, whereas at high nitrate it changes to
low-affinity mode. Here we are trying to examine how these biphasic responses are
regulated and maintained in a wide-range of variation in nitrate
availabilities. This question is addressed by means of signal transduction
model integrated with Boolean gate. I am also seeking structural explanations
by designing specific molecular dynamic (MD) simulation experiment.
NRT1.1 Regulation
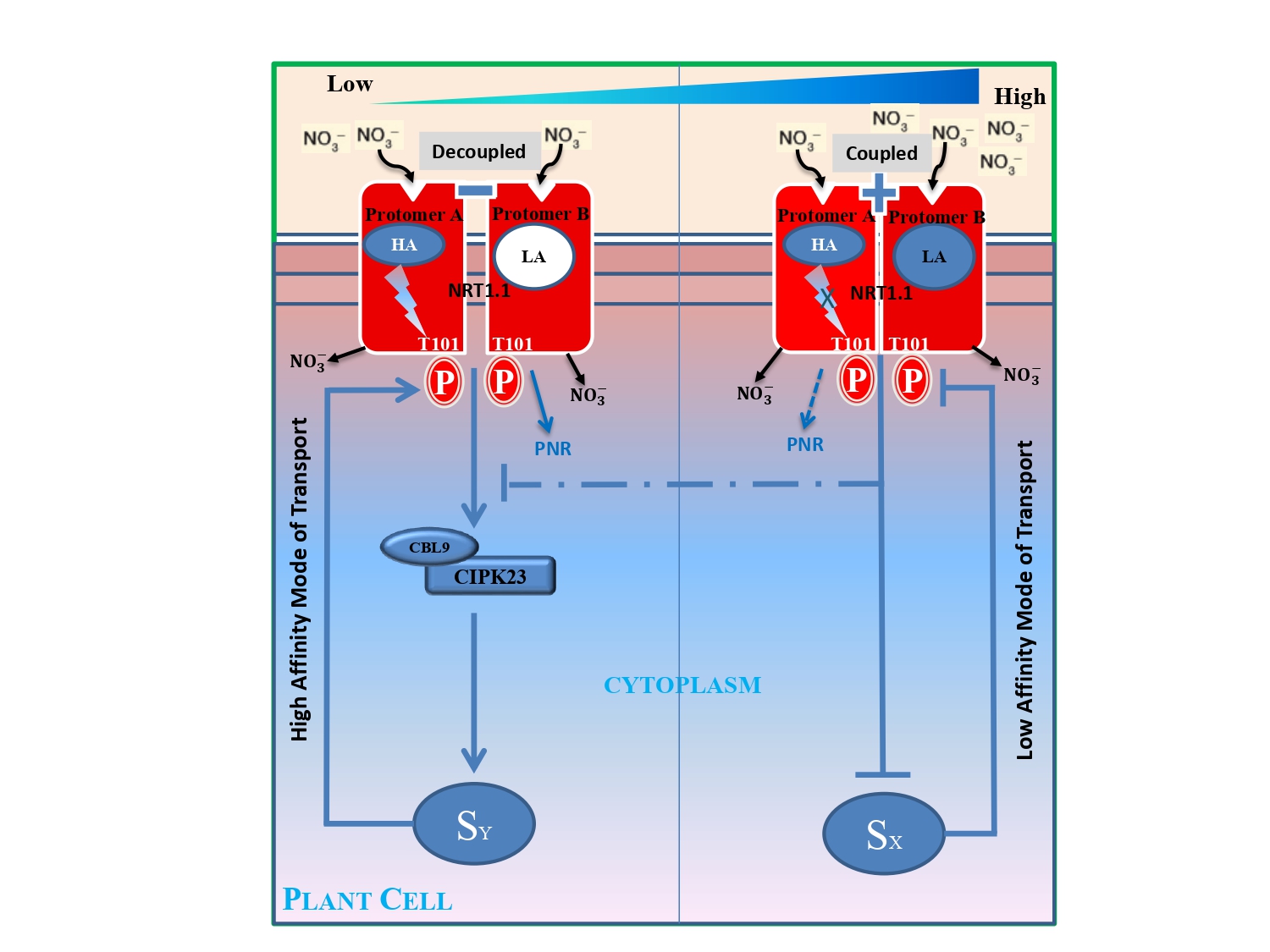
iScience (Cell Press) 2(2018): 41-50.The protomer A contains a high-affinity nitrate binding site, whereas the protomer B contains relatively a low-affinity binding site. Binding of nitrate ion triggers an allosteric communication between the binding site and the T101 site in protomer A that primes the T101 site for phosphorylation and responsible for activating the immediate downstream component of nitrate signaling CBL9.CIPK23 complex at low nitrate concentration. In contrast, such allosteric communication pathway is absent in protomer B. At low nitrate concentration, nitrate ion binds only at high-affinity site of protomer A and activates the CBL9.CIPK23 complex. At high-nitrate concentration, nitrate binds to both the sites of protomer A and protomer B and then continuously inhibits the activity of CBL9.CIPK23 complex along the increasing gradient of nitrate.